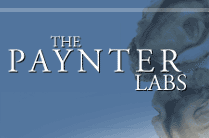
|
 |

  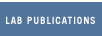 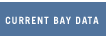   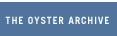 

|
 |
A Genetic Analysis of Oyster Recruitment Patterns in Chesapeake Bay
Problem Statement
Over the course of the last century, fishing pressure and disease have combined to reduce oyster populations to1% of the original standing crop. Because increasing sedimentation and anoxia have probably destroyed much previously suitable habitat for oyster settlement, and given that fertilization efficiency is strongly correlated with proximity and density of oysters (Paulis and Paynter, 2001), remnant oyster populations in Chesapeake Bay are probably fragmented to a much greater degree than ever before. If so, demographic isolation of sub-estuaries may exist on a time scale relevant to restoration efforts (ten years).
There is strong support in the mid-Atlantic region for Cheasapeake Bay oyster restoration with the goals of improving the ecological health of the ecosystem and building a more productive fishery (Chesapeake 2000 Agreement). A fundamental strategy in this effort is the delineation and protection of permanent sanctuaries where oysters can grow to an age at which they are highly fecund. Construction of artificial reefs is underway in Maryland and Virginia to provide crucial habitat, and these reefs are being manually seeded with juvenile or adult oysters.
Other than disease resistance, the most important population processes affecting oyster recovery are the dispersal and recruitment of juveniles into the population. Unfortunately, basic knowledge about the geographic scale and temporal heterogeneity of oyster recruitment is still lacking. Research efforts to identify sources of larval recruitment and explain huge spatial and temporal variation in recruitment observed over the last century have not provided general answers (Kennedy, 1996). Intuition built from a knowledge of oyster larval biology motivates arguments for broad dispersal distances and an ÔopenÕ demography (citation?). In contrast, recent experiments associated with reef restoration efforts have led to the conclusion that a large proportion of recruitment is local (Mann and student; unpublished data from the Chesapeake Bay Foundation and J. Wesson at Virginia Marine Recources Commission). Thus, basic questions remain about the extent and temporal consistency of local recruitment (i.e., self-recruitment of a reef versus transport from distant reefs). There is little doubt that oyster recruitment processes are dynamic and highly variable in both spatial and temporal dimensions. The goal is to empirically determine the geographic scale over which recruitment typically occurs and to estimate the local effective population size. The effective population size may be much lower than the census size for a given recruitment neighborhood if there is a large variance in reproductive success, and this has implications for temporal variability, inbreeding, and the efficacy of different restoration strategies.
Genetics has been one of the tools used in attempts to study these patterns, but the resolution of data and methods of analysis have been inadequate for the task (Buroker 1983; Brown and Paynter 1991). Recently developed high-resolution genetic markers and complementary analytical methods now make genetics one of the most powerful approaches to studying population processes on an ecological time scale, particularly in marine systems. The main advances that fulfill this potential are the availability of neutral DNA markers with high mutation rates (microsatellite markers) and methods of sampling and analysis that can quantify contemporary processes even in non-equilibrium populations.
As a first step toward describing the scale of oyster recruitment with a genetic approach, we were funded to conduct a single year (2002-2003) survey of genetic variation at several spatial scales in Chesapeake Bay oyster spat (young-of-the-year) and adults. This preproposal summarizes the very preliminary results of our 2002 effort and argues for continued funding to the sample and analyze oyster recruitment across several generations. This request is partly in response to comments from proposal reviewers that a single year study does not adequately sample the typical heterogeneity in Chesapeake oyster recruitment patterns. This is certainly true; only by comparing patterns of gene flow and self-recruitment across multiple years can we hope to measure the temporal scale of variability in these processes. For example, the higher salinity of Maryland waters during drought years may simply increase the magnitude of spawning and/or settlement, but it is also possible that different (upriver) populations contribute disproportionately during drought. A three year series of annual samples will only begin to represent the true extent of temporal heterogeneity, but it will provide a crucial baseline of data and analyses that can (1) provide meaningful estimates of the geographic scale for recruitment neighborhoods, and (2) provide focus to more extended monitoring schemes designed to evaluate restoration progress.
Genetics tools for understanding recruitment ecology
Highly variable genetic markers can be informative about recruitment in two ways. First, as selectively-bred disease resistant oysters are used in restoration plantings, genetically marked populations become available and their larvae can be distinguished from the background ÔnaturalsÕ. This type of local ÔexperimentÕ also provides opportunities to measure the extent of interbreeding and introgression from disease-resistant strains to natural oysters. These disease-related questions are being addressed with local sampling as part of a study funded by the Oyster Disease Research Program. The second use of genetics to understand recruitment is less direct, but uses patterns of allelic variation within and among populations to infer patterns gene flow on a broader range of geographic scales. In addition, temporal comparison of cohorts provides the most powerful estimates of reproductive variance and effective population size in contemporary populations, for example in the Pacific oyster (C. gigas; Li and Hedgecock, 1998).
Objectives
Preliminary Results from 2002 efforts: Using three microsatellite loci and sample sizes of N=50 spat per locality (plus 50 adults in one locality), our 2002 results for 12 localities already permit us to reject the panmixia hypothesis with high statistical confidence. Based on the following results, our primary goal is to extend our sampling of populations in order to analyze genotypes over a temporal window that can discriminate among several hypotheses:
Observation 1: significant genic differentiation of some spat samples from the others in patterns that seem unrelated to geographic proximity.
H1: Genetically differentiated source populations (adults)
H2: Adult panmixia in Chesapeake but occasional local recruitment with high variance in reproductive success
H3: Immigration of alleles from domestic stocks in certain locations (implies local recruitment)
Observation 2: Significant genic differentiation between spat and adults
local recruitment with high variance in reproductive success (low effective population size)
spatial heterogeneity in recruitment sources across generations
viability selection between spat and adult life stages
combination of these effects
Methods
Collections:
1. Whereas the first yearÕs sampling effort includes spat and adults, later year sampling will focus on spat. Young-of-the-year (n = 50 to 100) will be sampled at two to four sites within four different Chesapeake sub-estuaries throughout the Bay. Spat sampling localities will be as consistent as possible across years. Geographic comparisons will be used to test for macrogeographic panmixia. Spat samples will be compared across years and with adults to test for variance in reproductive success (in the case of adult panmixia) or extent of self-recruitment (if sub-estuaries are genetically differentiated).
2. Sample one sub-estuary more intensively (probably Tangiers). The same hypotheses as in (1) are being tested with these samples at micro- and meso-geographic scales.
All spat collections will be performed in the fall or early winter young of the year are easily distinguished from other cohorts.
Analysis
Population structure
Temporal variance and Ne
Seven Microsatellite loci (Brown et al. In press) will be assayed in each adult and spat sample using standard automated fluorescent genotyping procedures. These markers have a high mutation rate, creating high allelic diversity that increases statistical power for distinguishing closely related populations. For comparison with previous studies, standard population genetic indices of allele frequency variance among populations (e.g., Fst) will be calculated using GENEPOP (Raymond and Rousset 1995). However, a seven-locus genotype with highly variable markers will distinguish nearly every individual, and individuals can then be grouped according to the similarity of their genotype without making a priori assumptions about population boundaries or equilibrium status. This approach is referred to as an assignment test because individuals are ÔassignedÕ to a population based on the likelihood of its multilocus genotype being generated by that population (Rannala and Mountan 1997; Bernatchez and Duchesne 2000; Pritchard et al. 2000; Cornuet et al. 1999). Using this approach, the probability that an oyster from one river originated from an adjacent river, or the probability of exclusion (did not come from a certain river) can be calculated. The probability that spat came from adults on their home reef compared to adults at different distances away will be calculated. Isolation by distance will be tested by regressing genetic distance, as measured by Fst, against geographic distance (Slatkin, 1993; Hutchinson and Templeton, 1999). A Mantel test will be used to test for significance of the correlation. The ability to resolve the source of an individual depends on the degree of genetic subdivision and the number of markers. If more markers are needed for improved resolution, additional microsatellite loci are being developed and will be available for this study (K. Reece, Virginia Institute of Marine Science).
Anticipated Benefits
The current restoration plan is based on assumptions about the productivity of restored reefs and their ability to export recruits to surrounding habitat. The genetic analyses proposed here provide the only means to understand the population context within which restoration is being carried out, and to determine the optimum placement of limited reef material. Without this information, if the restoration efforts work well and achieve their goal, then everybody wins. If the restoration efforts fall short, however, these genetic data are an important means to understand why and to redirect efforts.
Facilities/Equipment Available and Required
Hare: All equipment needed to perform the genetic analysis is already available at UMD, including two 3100 genetic analyzers (Applied Biosystems) capable of processing 500 samples per day without multiplexing multiple markers in the same reaction. Materials required consist of chemicals and plasticware used in the genetic analysis.
Paynter: Field collecting equipment is available for Maryland waters. Boat charter will be required for Delaware and Virginia collections.
Personnel
P.I. Hare, genetic analysis, data analysis, publication
Co- P.I. Paynter, sample collection, publication
Technician for sample collection, processing, genetic analysis
|
 |
|
|
 |